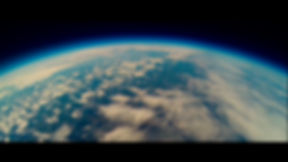

Description
FLASH-B
FLuorescenst Advanced Stratospheric Hygrometer for Balloon
Description of the instrument.
Abstract.
The FLASH-B hygrosonde (Yushkov et al. 1998, Yushkov et al. 2000) was developed at Central Aerological Observatory, Russia. The instrument is based on the fluorescent method (Kley and Stone, 1978; Bertaux and Dellanoy, 1978), which uses H2O molecules photodissociation at a wavelength l=121.6 nm (La - hydrogen emission) followed by the measurement of the fluorescence of excited OH radicals. The source of Lyman-alpha radiation is a hydrogen discharge lamp while the detector of OH fluorescence at 308 -316 nm is a photomultiplier run in photon counting mode. The intensity of the fluorescent light as well as the instrument readings is directly proportional to the water vapor mixing ratio under stratospheric conditions with negligible oxygen absorption.
This document gives detailed overview of the instrument’s design, describes its capabilities and application characteristics.
1. INTRODUCTION
Water vapor plays a unique role in atmospheric processes as a key chemical and radiative component. Traditionally, the global radiosonde network provides good vertical resolution of the lower troposphere humidity profiles. But due to significant problems arising at low temperatures and humidity, radiosondes give only rough estimates of water vapor concentration in the upper troposphere and tend to have very poor performance in the stratosphere. Satellite-borne and ground-based remote sensing provides valuable data on stratospheric water vapor concentration on a global scale, with the vertical resolution of the obtained profiles being, however, not better than 1-2 km. Improvement of the employed technique and creating new instruments become increasingly more important in meeting ever-growing requirements for the accuracy and resolution of atmospheric observation. The development of the remote techniques for studying the stratosphere also implies validation of the data obtained by in situ measurements.
A limited number of techniques are used for in situ measurements of the upper troposphere and stratosphere humidity. Among them, the fluorescent technique, offering high accuracy and fast response, has proved reliable. Based on this technique, an optical fluorescent hygrometer FLASH has been developed at Central Aerological Observatory.2,3 While FLASH instrument has been designed for use both on board balloons and high-altitude aircrafts, here we focus on the balloon modification of the hygrometer – FLASH-B.
Having undergone a number of major improvements since 1989, FLASH-B represents the low-weight and small-sized hygrometer integrated with Vaisala RS-80 radiosonde with the total weight of 1kg including power supply. The hygrometer can be easily integrated with ECC ozonesondes as well with other balloon-borne instruments for atmospheric research.
2. PRINCIPLE OF OPERATION
The fluorescent hygrometers for water vapor in situ measurements in the upper atmosphere with balloon, high-altitude aircraft, rocket were created and applied for atmospheric studies.1-8 The fluorescent method1 is based on the photodissociation of H2O molecules at wavelengths below 137 nm and subsequent fluorescent relaxation of the exited OH* radical produced. For Lyman-alpha dissociation of water vapor the process can be expressed as

The electronically excited OH* radical either fluoresces at 310 nm

or is quenching by collisions with air molecules

A photomultiplier with an interference filter measures the intensity of fluorescence which is signature of the parent molecules of . The intensity of fluorescence is obtained as
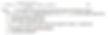
In determining the photon flux, absorption by oxygen has to be taken into account. At air pressure below 10hPa the quenching by air and oxygen absorption are negligible1 and the fluorescence intensity becomes


Thus, under condition with negligible oxygen absorption, the fluorescence gives a direct measurements of the atmospheric water vapor mixing ratio in this case. The coefficient in (6), representing optical properties is different for each hygrometer, thus a laboratory calibration of the instrument is necessary.
3. INSTRUMENT DESIGN
3.1 OVERVIEW OF THE INSTRUMENT
The FLASH-B instrument was developed at Central Aerological Observatory, Russia for balloon-borne water vapor measurements in the upper troposphere and stratosphere (Yushkov et al.,1998, Yushkov et al., 2000). The instrument is based on the fluorescent method (Kley and Stone, 1978; Bertaux and Dellanoy, 1978), which uses H2O molecules photodissociation at a wavelength λ < 137 nm followed by the measurement of the fluorescence of excited OH radicals. The source of Lyman-alpha radiation (λ =121.6 nm) is a hydrogen discharge lamp while the detector of OH fluorescence at 308 -316 nm is a HAMAMATSU R647-P photomultiplier run in photon counting mode with an narrowband interference filter for selecting the fluorescence spectral region. The intensity of the fluorescent light sensed by the photomultiplier is directly proportional to the water vapor mixing ratio under stratospheric conditions (30 – 150 hPa) with small oxygen absorption (3% at 50 hPa).
The precursor of FLASH-B instrument, optical hygrometer (Khaplanov et al., 1992) designed for use onboard both stratospheric balloons and rockets had a weight of about 5 kg. It participated in EASOE field campaign in 1991/92 (Khattatov et al, 1994) as well as in Arctic field balloon campaign in Russia.
The modified version of the optical hygrometer named FLASH-B (Fluorescent Advanced Stratospheric Hygrometer for Balloon) had significantly reduced dimensions and weight. It was successfully applied for water vapor measurements on board of long and short duration balloons (Yushkov et al., 2001). Based on this experience the recent version of FLASH-B integrated with Vaisala RS-80 radiosonde has been developed for regular balloon soundings.
The instrument uses the open layout [Khaplanov et al., 1992] where the optics is looking directly into the outside air. This arrangement is suitable only for nighttime measurements with a solar zenith angle larger than 980, at which sun light no longer reaches the detector. The co-axial optical layout allows reducing the size of the instrument to 106x156x242 mm with a total weight of 0.980 kg including batteries. The sketch of the hygrometer’s design is shown in Fig.1.

Fig. 1 Hygrometer design. 1 – kovar mounting, 2 – hydrogen lamp, 3 – photomultiplier, 4 – electronic unit, 5 – convex-concave lens, 6 – biconvex lenses, 7 – connecting tube, 8 – thread bushing, 9 – lamp housing, 10 – window-filter of the lamp, 11 – interference filter, 12, 13 – screw, 14 – teflon ring
The accuracy of the FLASH-B instrument is determined by the calibration error estimated as 4% in the 3 – 100 ppmv range. The measurement precision is 5.5% calculated for 4 seconds integration time at stratospheric conditions. The total uncertainty of the measurement is less than 10% at the stratospheric mixing ratios greater than 3 ppmv increasing to about 20% at mixing ratios less than 3 ppmv.
The source of vacuum UV (VUV) radiation used in the FLASH-B instrument is a hydrogen glow-discharge lamp filled with a mixture of hydrogen and helium at the total pressure of 10 hPa. Unlike the more sophisticated hygrometers based on the fluorescence technique, FLASH-B doesn’t use VUV photon flux control. However the hydrogen glow-discharge lamps used in the FLASH-B instrument have been proved to have very stable intensity of the Lyman-alpha emission over both operation and storage time. Every lamp is subjected to continuous laboratory tests for stability of the emission intensity which is checked before the flight. The VUV light sources containing the mixture of hydrogen and helium are known to have the stray helium line emission which overrides the spectrum of hydroxyl fluorescence and thus may cause spurious signal from backscattering of this emission. The FLASH-B instrument uses the hydrogen lamp in which the 270-320 nm emission is suppressed by a special window-filter. This window-filter is made using monocrystalline magnesium fluoride with an absorbing layer vacuum-deposited on its inner surface. In this way, up to 50% transmission at the 121.6 nm line and selective absorption at 300 nm are achieved. In addition the instrument uses the narrowband interference filter centered at 310 nm with 8 nm bandwidth and out-of-band extinction of 10-5 thus reducing the possible effect of the stray light backscattering.
The background signal caused by the night sky emissions in the absence of fluorescence light is detected using lamp modulation with 1 kHz square wave with 1/16 duty cycle and synchronous demodulation of the signal received. The background signal is detected while the lamp is off and then subtracted from the fluorescence signal.
The FLASH-B hygrometer is not an absolute instrument for water vapor measurements. Every hygrometer has to be calibrated in the laboratory before the flight. The laboratory studies showed that calibration coefficients remain constant in time. The lamp intensity, being the most influencing factor for calibration, is checked directly before the flight.
3.2 OPTICAL DESIGN
In fig. 2 the optical layout of the hygrometer is sketched. A system of three lenses is concentrically arranged around the lamp. The lenses are made from U-Viol glass US-49. The front lens has a diameter 50 mm and is sealed to the lamp body. The analyzed volume is located outside the instrument 24 mm off the window of Vacuum UltraViolet lamp (VUV). This separation largely prevents the effect of the desorption of water vapor (contamination) from the instrument's technological surfaces and communication lines, inherent in closed-type hygrometers using aspiration system. Use of the circular lenses provides the effective convergence of fluorescence from a large angle and thus increases the sensitivity of the instrument. However this compact open layout is suitable only for nighttime measurements with a solar zenith angle larger than 98o, at which sun light no longer reaches the detector.
After passing the interference filter with bandwidth 306 nm the fluorescence light hits the cathode of the photomultiplier(PMT). The photomultiplier is the HAMAMATSU R647P run in photon counting mode with bialkali cathode. This PMT is used for detecting the radiance in the spectral region 300-650 nm and has nominal sensitivity 1.8·105 A/Wt. The interference filter centered at 310 nm with 6 nm bandwidth and out of band extinction of is used to select the spectral region coincident with the emission from the upper rotational levels of the (00) band of the AX system of OH. Filter is designed as a system of multilayer (35 layers) dielectric hafnium and silicon sheets on a quartz substrate. Use of these materials allows producing filters with high transmission (not less than 50 %). The filter design ensures performance stability and safety from mechanical damage. The relative temperature dependence of the filter's transmission line shift is small and determined by magnitude. The manufactured filters afford the transmission of the most intensive part of a fluorescence signal.
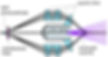
Fig .2 Schematics of the optical arrangement of the FLASH-B instrument.
3.3 HYDROGEN LAMP
Hydrogen lamps were used as sources of vacuum ultraviolet radiation for dissociation of water vapor molecules in reaction (1). In the hydrogen lamp, a source of quanta with energies of 10.2 eV is an atomic hydrogen gas discharge at Lyman line 121.6 nm. An oxygen spectral window at this line provides the most effective generation of excited hydroxyl radicals within the volume where fluorescence is being registered. This lamp refers to sources using hollow cathode radiation. The mechanical structure of this lamp is schematically shown in Fig. 3.

Fig.3. Hydrogen lamp.

Fig.4. The transmittance of monocrystalline magnesium fluoride window of hydrogen lamp without filter (1) and with filter (2) for selective absorption at 300 nm.
The lamp is filled with a 10% mixture of hydrogen and helium at the total pressure being 10 hPa. Due to high ionization energy and helium excitation (up to 34 eV), rather high-energy speed distribution of electrons is established in a gas discharge. This favors the formation and predominant excitation of atomic hydrogen in the VUV range, with the quanta energies of 10 to 15 eV. The symmetry of the electrical field, formed by the electrode structure of the lamp, conditions the concentration of ions in the cathode cavity. This provides the high degree of isolation of atomic and ionized hydrogen from the glass walls of the fixtures and the bulb. Estimation of the lamp radiation intensity at a 2-5 mA current and a 1 W input power has demonstrated that up to 80% VUV radiation flux is emitted at a 121.6 nm line, which amounts to about quanta per second. The lamp window-filter is made using monocrystalline magnesium fluoride, with an absorbing layer vacuum-deposited on its inner surface. In this way, up to 50% transmission at a 121.6 nm line and a selective absorption at 300 nm are achieved. Figure 4 shows transitivity values for a window made of magnesium fluoride (curve 1) and a window-filter (curve 2). The laboratory studies have shown that the temperature drift of the lamp does not exceed 0.016% / 0C.
3.4 ELECTRONIC DESIGN
The electronic part, shown in Fig.4. The electronic based on fast microcontroller with ARM TDMI core witch keep all process in hygrometers parts.
Microcontroller provides 1 kHz lamp modulation signal, witch control Lamp Driver for Lamp flashing. Using internal DAC, microcontroller can set up and keep up the Lamp current value very precisely. Because the Lamp is biggest power consumer, the Lamp current value is balance between sensitivity and battery power.
The second DAC is set up photomultiplier (PMT) voltage value in PMT power supply. Internal ADC measure the PMT voltage for keep up the value in 1 V precision in all operating conditions.
Microcontroller is set up inside temperature and control of heater, for keep the instrument temperature the same as under calibration during all flight.
The output signal of PMT is impulses from fluorescence photons are collected in to internal counter of microcontroller. Microcontroller has two counters: for fluorescence light and for background light. When the lamp is on, the counter is counting the fluorescence photons, and when the lamp is off, the counter is subtracting dark counts. The difference of these counters values is raw hygrometer data.
Hygrometer has the pressure sensor onboard. The PMT is switch on by pressure sensor output on 2 km altitude, for avoidance the PMT go blind by big ground lights during launch. In descending below 2 km the Lamp and PMT are switch off for safe landing for recovery.
Microcontroller have several interfaces for connecting to different radiosondes. For communicate to Vaisala RS92 radiosonde it has interface converter from UART to SPI port. The hygrometer data going to RS92 radiosonde by digital interface and download to the ground station in composition radiosonde data. Using UART interface, hygrometer can work with other radiosonde system, like Meteolabor SRS-34 (Switzerland), Meisei RS-06G (Japan), Intermet (USA).
Power consumption of hygrometer is 0,5 A from 12 V. For standard 4 hours flight is needs 4 Lithium batteries size C with around 4 A/h capacity, for example SAFT LSH 14.
Maximum weight of hygrometer with insulation box and batteries no more than 1 kg.
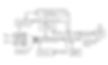
Fig.4. The electronic part
4. CALIBRATION OF THE HYGROMETER
For calibration a laboratory facility capable of simulating atmospheric conditions is used. In particular, the large range of water vapor mixing ratios (1-1000 ppmv), pressure from 1000 to 3 hPa and temperature down to 190 K can be generated by the calibration setup.
The calibration procedure is performed as follows: after purging the system with dry air for 1 – 2 hours, the airflow boosted by the compressor is dried passing a silica gel dehumidifier and then divided into two branches of which one is moistened in a H2O bubbler. Via the two flow controllers the airflows of both branches are mixed together in a homogenizer producing variable H2O mixing ratios.
After passing the homogenizer the mixed airflow is divided so that one branch flows through a commercial reference dew point hygrometer MBW 373L to determine the H2O mixing ratio, while another branch goes to the stainless steel chamber cooled down to 210 K in the low temperature freezer. The fluorescence hygrometer is flanged to the chamber via vacuum caulking. The pressure in the chamber is reduced by the vacuum pump to 50 hPa. The calibration is started with the lowest H2O mixing ratio, which is then increased stepwise. Every calibration point is measured for about 15 minutes.
The calibration fit function is linear in the pressure range of 30 – 150 hPa and water vapor mixing range of 1 – 300 ppmv. At higher pressures the VUV absorption by oxygen and water vapor is taken into account. The lamp stray light being constant doesn’t affect the calibration since the calibration coefficients are determined as the slope of the regression line.
The total uncertainty of the calibration is determined by the following factors: uncertainty of the frost point measurement (0.1 K), uncertainty of the temperature dependence of the water vapor partial pressure, error in pressure determination, error accounting for inconsistency of the air sampled by the reference dew point hygrometer and the air inside the chamber, instability of the VUV intensity of the lamp. The total relative error of the calibration amounts to 4%.
For the calibration of the hygrometer, two independent procedures are currently used. One of them is the calibration in vacuum tank in pressure range mbar, where the hygrometer signal and water vapor partial pressure are directly proportional. The main purpose is to obtain ratio of the hygrometer signal and water vapor pressure in this pressure range. This ratio is the coefficient in formula (5). This ratio obtained is substituted into the formula (6) together with known coefficients A and .
For accurate calibration of optical hygrometer the vacuum system developed in Central Aerological Observatory has been used. The hygrometer is attached to the main vacuum tank (0,5 ), where pressure is measured by the ionization gauge. Total uncertainty of pressure measurements by ionization gauge due to nonlinearity, hysteresis, and non-repeatability in this pressure range is less then 10%.. Before the calibration the system is evacuated by absorption and electrodischarge pump down to mbar. Then the water vapor is fed from the water container into the small tank to obtain pure water vapor at pressure about 0.1 mbar. in this tank. During the calibration, a constant water vapor flow from the small into the large tank is achieved. Since the pressure in the main tank is produced by pure water vapor, the ionization gauge measures water vapor pressure. Typical time of calibration, when pressure changes fromup to mbar. is 40 seconds, while the pressure and signal are measured each second. To reduce the calibration error, this procedure is repeated 10 times.
Another technique is calibration in a “cold chamber” in pressure range 10-100 mbar at water vapor mixing ratios 5-100 ppmv. The hygrometer is attached to a steel chamber with a cooled copper ring with 50mm diameter in it. The ring temperature can be changed within +20..-120 C temperature range, and the inner side of the ring covered by thin layer of ice. The temperature of the ring is measured by three thermistors.
As it follows from (6), the hygrometer readings are proportional to the water vapor mixing ratio in the pressure range 10-200 mbar. The water vapor mixing ratio is defined by the ice temperature and total pressure in the calibration chamber. During the calibration, the temperature of the ring is varied at constant pressure. In this case, we directly determine the dependence between the hygrometer readings and water vapor mixing ratio for the upper troposphere and stratosphere conditions.
The total accuracy as it follows from (6) depends mainly on the accuracy of calibration coefficient as well as on the accuracy of hygrometer's signal measurements U (2%). The accuracy of calibration coefficient is determined by the precision of low pressure measurement by Russian VIT-2 ionization vacuummeter (8%). The total accurace is better than 10%.
5.FLIGHT LAYOUT
The optical fluorescent hygrometer looks like cylinder with the diameter 110 mm and length 200 mm. The total weight of hygrometer without power supply is about 2kg. It is used with the VAISALA RS-80 radiosonde and can be readily integrated with other flight instruments for in situ atmospheric trace measurements (ECC ozonesonde, backscattersonde, optical counter and so on). Relatively small rubber TOTEX TX-3000 balloon or plastic RAVEN balloon can be used for flight experiments. To avoid the contamination from balloon the increasing up to 40 m the distance between the hygrometer and balloon is provided by the downreel. The flight position of the hygrometer is horizontal.
6. CONCLUSIONS
1. A compact instrument has been successfully upgraded to carry out high-resolution night-time upper tropospheric and stratospheric water vapor balloon measurements.
2. Using coaxial optical scheme, electrical modulation of a hydrogen lamp with a frequency of 1 kHz, PMT in photon counting mode, synchrodemodulation of the fluorescent signal is the basis of the new version of the open-type hygrometer without an aspiration system.
3. The hygrometer can be used together with the Vaisala RS-80 radiosonde, as well as with the ECC ozonesonde.
4. The weight of the hygrometer with Vaisala RS-80 radiosonde and batteries doesn’t exceed 3 kg . Therefore, a TOTEX ТХ-3000 balloon can be used, which extends the use of the hygrometer for satellite-borne and ground-based devices validation.
5. The total accuracy mainly depends on the calibration procedure and does not exceed 10%. 3 sec averaging of the flight raw data provides a possibility to study the fine structure of the observed vertical water vapor profile.
7. ACKNOLEDGEMENTS
We wish thank to Dr.H.Nakane(National Institute for Environmental Studies, Japan) for fruitful discussions and help in this work
8. REFERENCES
1. D.Kley, E.Stone,"Measurements of water vapor in the stratosphere by photodissociation with Ly-a (1216 A) light", Rev.Sci.Instrum., vol 49, No 6, pp 691-697, 1978
2. Yushkov V., A. Lukjanov, M. Khaplanov, et al. “Optical fluorescent hygrometer for water vapor concentration measurements.” Air Pollution And Visibility Measurements, edited by P. Fabian, V. Klein, M. Tacke, K. Weber, and C. Werner, 2506, 783-794, 1995:
3. Merkulov S. and V.Yushkov. “Fluorescent Airborne Stratospheric Hygrometer (FLASH) and some results of the Airborne Polar Experiment (APE) during 1996/1997 winter period”. Proc. Of the fourth European symposium. Edited by N.R.P.Harris, I.Kilbane-Dawe and G.T.Amanatides, pp. 474-477.
4. Yushkov V., Dorokhov V., Zvetkova N., Lukyanov AQ., Zaitcev I., Merkulov S., and Nakane H.”A comparison of ozone, hygro and thermal tropopause heights over Yakutsk in 95/96, 96/97 winter-spring period”. ”. Proc. Of the fourth European symposium. Edited by N.R.P.Harris, I.Kilbane-Dawe and G.T.Amanatides, pp.231-234
5. 3. E. Weinstock, J.Schwab, J.B.Nee, M.Schwab, J.Anderdson, "A cryogenically cooled photofragment fluorescence instrumentfor measuring stratospheric water vapor", Rev.Sci.Instrum, vol 61, No5, pp 1413-1432, 1990
6. U.Morchel, E.Klein, D.Kley, U.Schmidt, "A new balloon borne stratospheric hygrometer", Proceedings 10 th ESA sympozium on European rocket and balloon programs and related research, Mandelieu-Cannes, France, 27-31 May, pp 201-206, 1991
7. F.Goutail, J-P.Pommereau,"Stratospheric water vapor in situ measurements from infra-red montgolfier", Adv. Space Res., vol 7, No 7,pp 111-114, 1987
8. Kelly K.,"Lyman /OH fluorescence hygrometers for the Stratospheric-Tropospheric Exchange Project" Moisture and Humidity: Proceedings of the 1985 Symposium on Moisture and Humidity, pp. 463-465, Instruments Society of America, Research Triangle Park, N.C. 1985.